Browse our range of datacentre HDDs
2.5in SATA HDDs >
2.5in SAS HDDs >
3.5in SATA HDDs >
3.5in SAS HDDs >
Datacentre SSD and HDD Buyers Guide
What are Datacentre Storage Drives?
Datacentre storage drives are used in servers, storage appliances and NAS boxes to store your organisations data, so reliability is key characteristics to ensure your data is kept safe. Broadly speaking there are two main types of enterprise storage drive - HDDs (hard disk drives) where data is stored magnetically on spinning platters and SSDs (solid state drives) which have no moving parts and data is written to memory cells.
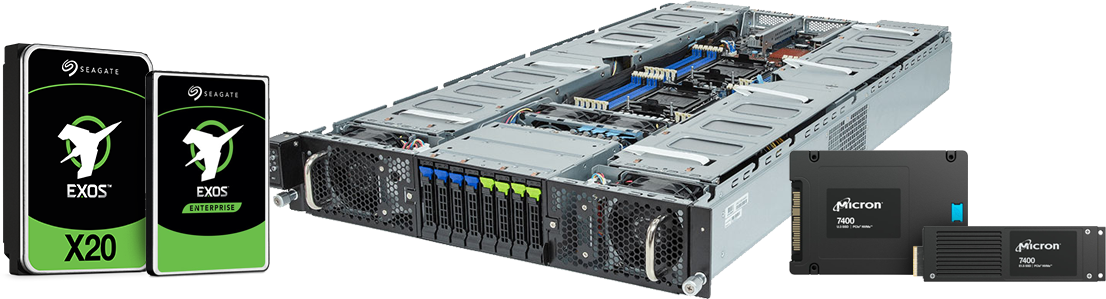
Although SSDs are a much newer technology and are much faster, HDDs still have a place, especially in business-critical devices such as servers - where the consideration isn’t just about performance, but with getting the right type of storage for the data usage and the degree of cost to store it. In this guide we’ll run you through all the details of HDDs and SSDs, how they work, the pros and cons of each and the scenarios where each is best suited. If you are looking for either HDDs or SSDs for consumer, prosumer, or NAS use, you can learn more by reading our HDD Buyers Guide , SSD Buyers Guide or NAS Buyers Guide.
What Datacentre Storage Drives Should I Choose?
In most cases the type of workloads you are dealing with may dictate to a large degree what kind of storage drive to choose - this may be due to size, cost, performance or a combination of these.
Datacentre Drives for Servers
In a server it is common that the OS to sit on separate drives to the applications and data, so with this in mind an M.2 SSD is ideal. Firstly large capacities are not required for the OS, and secondly it keeps traditional drive bays free for data storage. It is also worth considering a second SSD to provide redundancy and failover for the OS. For data storage it again depends on usage - large file sizes and/or regular access requirement will benefit from SSDs. As a typical server chassis will take anywhere from eight to 24 drives, then the choice of 2.5in or 3.5in drives does play an important part.
Datacentre Drives for Storage Servers
Storage servers are a little different to servers designed for compute - it is still common that the OS will sit on separate drives to the applications and data, so with this in mind a pair of M.2 SSD is ideal. For the storage drives, the type and number will depend on the amount of data you have and how much you expect it to increase - SSDs are faster, but more expensive and HDDs offer larger capacities. Sometimes it may be that a mix of both best suits the data access needs. For instance, NVMe SSDs can be deployed for hot data, SATA SSDs for warm data and HDDs for cold data. As a typical storage server chassis may take up to 60 drives is then choice of 2.5in or 3.5in drives does play an important part too. Lastly, for very dense deployments there is the option of EDSFF drives.
Datacentre Drives for NAS Appliances
NAS (Network Attached Storage) appliances are a little different. Firstly, the OS is inbuilt on the system, so you’re only looking for data storage drives. A NAS device is a good way to share files in a small office environment and can be very cost effective for large capacities using 3.5in HDDs - always look out for vibration resistant HDDs if you have greater than four drive bays in your NAS, as the lateral vibration produced by multiple spinning disks affect performance and drive lifespan. However, we would only recommended SSDs if you are directly connected to the NAS – the extra speed of SSDs will be lost over most network connections. In most cases high capacity 3.5in HDDs are the way to go. You can learn more about storage drives for NAS boxes by reading our dedicated NAS buyers guide.
Datacentre Storage Drive Sizes and Interfaces
Now we’ve mentioned broadly the type of drive to look for depending on your device, let’s look at the difference between them in more detail. There are a number of SSD types available, depending on their physical size and how they connect to your device. Although it’s worth starting with the interface, you’ll also need to check within your system to understand what drive bays are available - both size and in number if you are looking to use multiple drives.
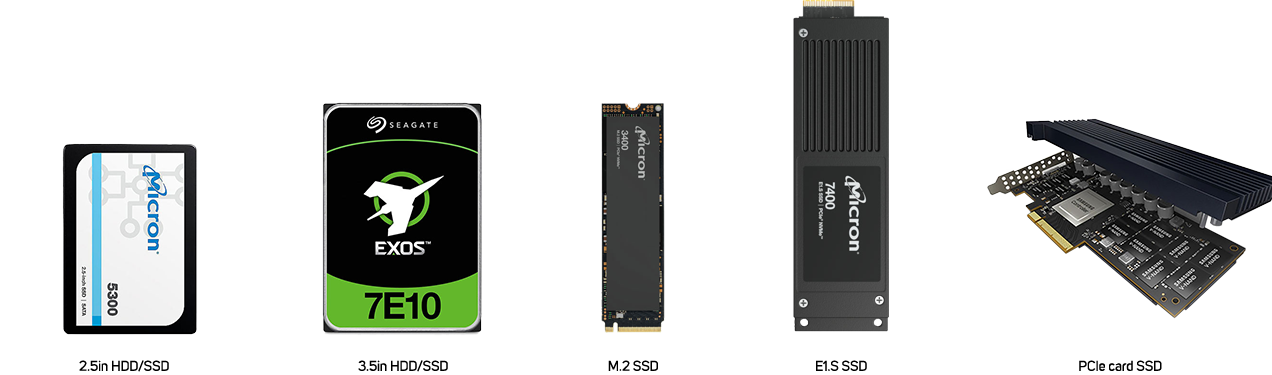
The various drive sizes can also have different types of interface that will affect performance, as well as the number of drives that can be installed within any given device. The tabs below give a summary of the performance of each interface and which of the interface options are available in each drive size.
SATA
SATA is a very common type of interface for both HDDs and SSDs. There have been several generations of SATA, the latest being SATA-III, each delivering increasing throughput speeds - the maximum now being 600MB/sec.
3.5” HDDs / SSDs | 2.5” HDDs / SSDs | M.2 SSDs | E1.S SSDs | PCIe card SSDs |
---|---|---|---|---|
yes |
yes |
yes |
no |
no |
SAS
SAS is another common interface for HDDs and SSDs but usually seen in higher end devices. Like SATA there have been several generations – the latest SAS-4 delivers 2.4GB/sec. SATA drives will work attached to a SAS controller but not vice versa.
3.5” HDDs / SSDs | 2.5” HDDs / SSDs | M.2 SSDs | E1.S SSDs | PCIe card SSDs |
---|---|---|---|---|
yes |
yes |
no |
no |
no |
M.2 NVMe
The M.2 is a slot designed for SSDs that plug directly into the motherboard, offering a choice between either an M.2 SATA SSD or an M.2 NVMe SSD. Due to its small size M.2 drives tend to be lower capacity than 2.5in SSDs. As M.2 SATA drives still use the SATA-III interface then maximum performance is 600MB/sec, whereas an M.2 NVMe SSD would benefit from speeds up to 15.8GB/s using four lanes of PCIe 5.0.
Beware that while most M.2 drives are 80mm long, some high capacity drives are 110mm long and will not fit in all motherboards. Check your server manual before buying.
3.5” HDDs / SSDs | 2.5” HDDs / SSDs | M.2 SSDs | E1.S SSDs | PCIe card SSDs |
---|---|---|---|---|
no |
no |
yes |
no |
no |
U.2 / U.3 NVMe
U.2 NVMe SSDs directly connect to the PCIe bus, however a cable is required rather than it being an on-board slot like the M.2 connector. It delivers the same 15.8GB/s performance using PCIe 5.0, though the U.2 interface supports SSDs in the more common 2.5in format so capacities can be bigger than more compact M.2 SSDs.
U.3 is a new interface standard that is an evolution of U.2, the main benefit being that the disk backplane inside the server chassis can carry SATA or NVMe signals through one physical connector and one cable - this results in fewer cables inside the server which in theory means lower server cost.
3.5” HDDs / SSDs | 2.5” HDDs / SSDs | M.2 SSDs | E1.S SSDs | PCIe card SSDs |
---|---|---|---|---|
no |
yes |
no |
no |
no |
E1.s NVME
E1.S NVMe SSDs directly connect to the PCIe bus, however a cable is required rather than it being an on-board slot like the M.2 connector. It delivers the same 15.8GB/s performance using PCIe 5.0. E.1S drives are considerably smaller than 2.5in U.2 or U.3 drives, allowing more drives to be installed in the same size server.
3.5” HDDs / SSDs | 2.5” HDDs / SSDs | M.2 SSDs | E1.S SSDs | PCIe card SSDs |
---|---|---|---|---|
no |
no |
no |
yes |
no |
PCIe Card
E1.S NVMe SSDs directly connect to the PCIe bus, however a cable is required rather than it being an on-board slot like the M.2 connector. It delivers the same 15.8GB/s performance using PCIe 5.0. E.1S drives are considerably smaller than 2.5in U.2 or U.3 drives, allowing more drives to be installed in the same size server.
3.5” HDDs / SSDs | 2.5” HDDs / SSDs | M.2 SSDs | E1.S SSDs | PCIe card SSDs |
---|---|---|---|---|
no |
no |
no |
no |
yes |
Datacentre HDDs vs Enterprise SSDs
The below table shows a feature comparison of datacentre HDDs and SSDs, highlighting where the advantages and disadvantages lie.
HDD | SSD | Comparison | |
---|---|---|---|
Performance | Hundreds of MB/sec | Thousands of MB/sec | SSDs are much faster |
Access Times | 5-8ms | 0.1ms | SSDs have almost no latency |
Reliability | 2-5% failure rate | 0.5% failure rate | SSDs are much more reliable |
Resilience | Susceptible to vibrations | No moving parts | SSDs are much safer to install in a laptop |
Energy Use | 6-15W | 2-5W | SSDs are much more energy efficient |
Noise | 20-40dB | Silent | No noise from SSDs |
Capacity | Up to 26TB | Up to 15TB | HDDs provide more capacity |
Cost | £-££ | ££ - ££££ | SSDs are more expensive, especially at high capacities |
Although SSDs clearly have the edge over HDDs in most aspects, there are still specific uses where HDDs excel, dictated by their price to performance ratio. HDDs are much more cost-effective than SSDs - a key fact in a use case such as data archiving, where capacity is more important than access speed. This lower performance but cost effectiveness is due to how HDDs work, as shown below.
An HDD contains one or more spinning disks called platters. These platters have thousands of tiny segments, with the ability to be individually magnetised (1) or demagnetised (0). It is the sequence of 1s and 0s that store data. The magnetisation state of the platters is physically changed by an arm that passes over the platter and writes the data to a section of it. Once data is stored on the platter by way of magnetisation it will remain in this state even when the power is off and the platters stop spinning, until it is either written over with new data or deleted altogether. Over time HDDs have seen the capacity of a single platter increase and the number of platters increase resulting in larger and larger drive capacities.
As an HDD capacity gets larger there may be greater latency in retrieving data from it, as the arms physically have to trace a larger area of bytes distributed over multiple platters. For this reason HDDs come in variety of spin speeds have an impact on the rate at which data can be written and retrieved from the HDD - typical speeds range from 7,200rpm to 15,000rpm. It is also worth noting that as you fill up a HDDs with data its performance goes down, caused by data fragmentation as files are split over several sections of the drive depending on what space was available at the time of writing. When it comes to reading the data the arms have to find the fragmented sections of it and piece it together so it makes sense. This is one factor why using many smaller capacity drives can be better than single or fewer large ones. The below table highlight the different performances, costs and typical uses of speed types.
7,200rpm | 10,000rpm | 15,000rpm | |
---|---|---|---|
Capacity | High Capacity | Medium Capacity | Low Capacity |
Read / Write | Medium Performance | High Performance | Highest Performance |
Typical Device | Archiving | Server | Server |
Cost | ££ | £££ | ££££ |
HDDs, although cost effective for storage server use, pose a unique problem in that many drives situated next to each other spinning up to 15,000 times a minute create significant vibration that can cause server issues and shorten the drive lifespan. This is countered by datacentre and high-end NAS HDDs having vibration resistance features as standard.
Like an HDD, SSDs stored data is kept in place when there is no power to the drive. However, that is where the similarity ends – unlike HDDs which store data magnetically on spinning platters, SSDs store data on memory chips called NAND. This is where the term solid state comes, because there are no moving parts within the drive. As writing and reading from NAND doesn’t require a physical arm to access the data, performance is much faster. NAND is made up of transistors in columns and rows which can either conduct current (1) or don’t conduct current (0). When precise voltages are applied to the network of transistors (called cells) a pattern of 1s and 0s is formed to represent the data.
NAND memory can be classed in several types based on how many 1s and 0s can be stored in each cell. Single-Level Cell (SLC) NAND stores one bit - either a 1 or a 0 - per cell, whereas Multi-Level Cell (MLC) NAND stores two bits per cell. Triple-Level Cell (TLC) NAND stores three bits per cell and Quad-Level (QLC) NAND stores four bits per cell. Each of these types offer differences in maximum capacity, longevity, reliability and cost. Although an SSD contains no moving parts to fatigue, the cells within NAND do wear out through repeated Program (writing) / Erasing (P/E) cycles, although the drive controller will work to spread data evenly across the cells to create consistent wear across the whole capacity. The below table highlight the different performances, costs and typical uses of NAND types..
Quad level Cell
(QLC) |
Triple level Cell
(TLC) |
Multi level Cell
(MLC) |
Single Level Cell
(SLC) |
|
---|---|---|---|---|
Architecture | 4-bits per cell | 3-bits per cell | 2-bits per cell | 1-bits per cell |
Capacity | Highest capacity | High capacity | Medium Capacity | Lowest Capacity |
Endurance | Lowest Endurance (~1,000 cycles) | Medium endurance (~3,000 cycles) | High Endurance (~10,000 cycles) | Highest Endurance (~100,000 cycles) |
Use | Read | Read / Write | Write / Read | Write |
Typical Devices | Cost effective server | Mid-range server | High performance server | Critical storage |
Cost | £ | ££ | £££ | ££££ |
Data Security
When considering which SSD to buy it is vital to understand about how best to protect the data on your drives. This can be achieved in a number of ways using RAID technology. RAID stands for redundant array of independent disks and it is a way of spreading the data across multiple drives to remove the chance of a single point of failure. It works by blocks of data, referred to as parity blocks, being distributed across the multiple drives so that in the event of failure of any one drive the parity blocks can be used to retrieve the lost data and rebuild the array. RAID levels are categorised by number and their attributes vary with each type.
RAID 0
RAID 0 is the fastest RAID mode since it stripes data across all of the array’s drives and as the capacities of each drive are added together it results in the highest capacity of any RAID type. However, RAID 0 lacks a very important feature - data protection. If one drive fails, all data becomes inaccessible, so while RAID 0 configuration may be ideal for gaming where performance matters but data is not important, it is not recommended for storing critical data.
RAID 1
RAID 1 works across a maximum of two drives and provides data security since all data is written to both drives in the array. If a single drive fails, data remains available on the other drive, however, due to the time it takes to write data multiple times, performance is reduced. Additionally, RAID 1 reduces disk capacity by 50% since each bit of data is stored on both disks in the array. RAID 1 configurations are most commonly seen when mirroring drives that contain the operating system (OS) in enterprise servers, providing a back-up copy.
RAID 5
RAID 5 writes data across all drives in the array and to a parity block for each data block. If one drive fails, the data from the failed drive can be rebuilt onto a replacement drive. A minimum of three drives is required to create a RAID 5 array, and the capacity of a single drive is lost from useable storage due to the parity blocks. For example, if four 2TB drives were employed in a RAID 5 array, the useable capacity would be 3x 2TB = 6TB. Although some capacity is lost, the performance is almost as good as RAID 0, so RAID 5 is often seen as the sweet spot for many workstation and NAS uses.
RAID 6
RAID 6 writes data across all drives in the array, like RAID 5, but two parity blocks are used for each data block. This means that two drives can fail in the array without loss of data, as it can be rebuilt onto replacement drives. A minimum of four drives is required to create a RAID 6 array, although due to the dual parity block, two drives capacities are lost - for example if you had five 2TB drives in an array, the usable capacity would be 3x 2TB = 6TB. Typically due to this security versus capacity trade-off, RAID 6 would usually only be employed in NAS appliances and servers with critical data.
RAID 10
RAID 10 is referred to as a nested RAID configuration as it combines the protection of RAID 1 with the performance of RAID 0. Using four drives as an example, RAID 10 creates two RAID 1 arrays, and then combines them into a RAID 0 array. Such configurations offer exceptional data protection, allowing for two drives to fail across two RAID 1 segments. Additionally, due to the RAID 0 stripe, it provides users high performance when managing greater amounts of smaller files, so is often seen in database servers.
RAID 50
RAID 50 is referred to as a nested RAID configuration as it combines the parity protection of RAID 5 with the performance of RAID 0. Due to the speed of RAID 0 striping, RAID 50 improves upon RAID 5 performance, especially during writes, and also offers more protection than a single RAID level. RAID 50 is often employed in larger servers when you need improved fault tolerance, high capacity and fast write speeds. A minimum of six drives is required for a RAID 50 array, although the more drives in the array the longer it will take to initialise and rebuild data due to the large storage capacity.
RAID 60
RAID 60 is referred to as a nested RAID configuration as it combines the double parity protection of RAID 6 with the performance of RAID 0. Due to the speed of RAID 0 striping, RAID 60 improves upon RAID 6 performance, especially during writes, and also offers more protection than a single RAID level. RAID 60 is often employed in larger server deployments when you need exceptional fault tolerance, high capacity and fast write speeds. A minimum of eight drives is required for a RAID 60 array, although the more drives in the array the longer it will take to initialise and rebuild data due to the large storage capacity.
Systems that support RAID arrays will usually have a hot-swap capability, meaning that a failed drive can be removed from the array without powering the system down. A new drive is put in the failed drives place and the array rebuild begins - automatically. You can also configure a hot spare drive - an empty drive that sits in the array doing nothing until a drive fails, meaning that the rebuild can start without the failed drive being removed first.
It is also worth mentioning that multiple RAID arrays can be configured in a single system - it may be that RAID 1 is employed to protect a pair of SSDs for the OS, whereas multiple drives are protected by RAID 6 including hot spare drives too. Ultimately however, the RAID configuration(s) you choose need to be controlled, either by software on the system or additional hardware within it. Let’s take a look at the options.
Hardware RAID
In a hardware RAID setup, the drives connect to a RAID controller card inserted in a PCIe slot or integrated into the motherboard. This works the same for larger servers as well as workstations and desktop computers, and many external drive enclosures have a RAID controller built in. High-end hardware RAID controllers can be upgraded with a cache protector, these comprise a small capacitor which in the event of power loss keeps powering the cache memory on the RAID controller for as long as three years. Without a cache protector, data stored in the RAID controllers cache will be lost and could cause data corruption.
Advantages | Disadvantages |
---|---|
• Better performance, especially in more complex RAID configurations. Processing is handled by the dedicated RAID processor rather than the CPUs which results in less strain on the system when writing backups, and less downtime when restoring data
• Has more RAID configuration options including hybrid configurations which may not be available with certain OSes • Compatible across different OSes. This is critical if you plan to access your RAID system from say, Mac and Windows. Hardware RAID would be recognisable by any system |
• Since there’s more hardware, there’s more cost involved in the initial setup
• Inconsistent performance for certain hardware RAID setups when using SSDs • Older RAID controllers disable the built-in fast caching functionality of SSDs that are needed for efficient programming and erasing onto the drive |
You can learn more about RAID controllers by reading our dedicated Storage Controllers Buyers Guide.
Chipset RAID
Many AMD and Intel motherboard chipsets support some of the basic types of RAID, potentially negating the need for a hardware RAID controller.
Advantages | Disadvantages |
---|---|
• No additional cost - all you need to do is connect the drives and then configure them in the OS
• Modern CPUs are so powerful they can easily handle RAID 0 & 1 with no noticeable performance hit |
• You’re restricted to the RAID levels your motherboard chipset supports
• Performance hit if you’re using more complex RAID configurations • Limited performance and resilience compared to hardware RAID controller • If the motherboard dies you lose access to the RAID array |
Software RAID
The third and final type of RAID array is called software RAID and is when you use the operating system to create a RAID. Numerous operating systems support RAID, including Windows and Linux.
Advantages | Disadvantages |
---|---|
• No additional cost - all you need to do is connect the drives and then configure them in the OS
•Modern CPUs are so powerful they can easily handle RAID 0 & 1 with no noticeable performance hit |
• Software RAID is often specific to the OS being used, so it can’t generally be used for drive arrays that are shared between operating systems
• You’re restricted to the RAID levels your specific OS can support • Performance hit if you’re using more complex RAID configurations • If the OS dies you lose access to the RAID array |
Storage Drive Capacity
Although it is almost impossible to advise on the drive capacity you may require, as this will depend on the file types you have - text, audio, video or HD video - and the size and number of them, there are a few good rules of thumb to follow.
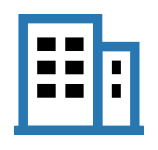
Small Office
For a small office a single server may be all that is required, so it may be the server and the storage capability for your organisation. In this case capacity may be the key so HDDs could be the most effective drives to choose. Additionally if everyone is accessing this shared resource over a standard 1GbE network, then access time would probably negate SSD speed anyway. If your data requirement grows a NAS can easily be added for dedicated storage and reducing the single point of failure on the server. Any drives for a business should be protected by RAID even if by simple mirroring.
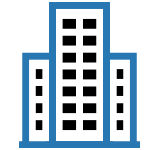
Large Organisations
Larger organisations are likely to have multiple servers and separate storage devices, so the drive capacities can be tailored accordingly. The servers providing critical functions should be equipped with SSDs, including those for front line storage as this is likely to be accessed by all employees. It is recommended that storage drives aren’t too large in capacity as this causes long rebuild times in the event of drive failure. For any archive storage large capacity HDDs are fine, as they aren’t accessed that much so cost effectiveness is key.
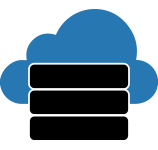
Cloud and vGPU
For servers providing virtualised graphics or cloud functionality, SSDs are also chosen for their speed and consistency, however it is advisable to ensure the sturdiest RAID protection setting are used as the data is only kept in the centralised location for all the virtual services. Again, smaller capacities are recommended to negate long rebuild times in the event of drive failure.
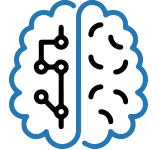
AI
Servers and storage concerned with providing data throughout to GPU-accelerators working on AI workflows usually require both low latency and high transfer rate drives as training models can generate large amounts of data very rapidly. These types of system are typically expensive as the highest capacity NVMe SSDs are recommended to deliver the performance and reliability required.
Ready to buy?
Browse our range of datacentre SSDs
2.5in SATA SSDs >
2.5in SAS SSDs >
M.2 NVMe SSDs >
U.2 NVMe SSDs >
U.3 NVMe SSDs >
E1.S NVMe SSDs >
PCIe card SSDs >
Alternatively if you have any further questions you’d like answering about enterprise storage drives and their installation, don’t hesitate to call one of our friendly advisors on 01204 474747.
Also, don’t forget if you want information on consumer or prosumer HDDs, SSDs, or NAS-grade drives, you can learn more by reading our
HDD Buyers Guide
,
SSD Buyers Guide
or
NAS Buyers Guide.